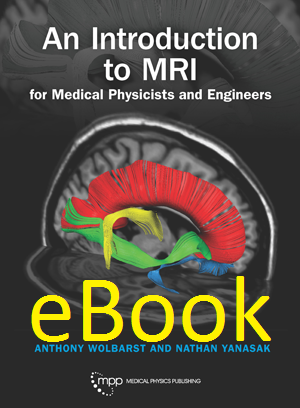
An Introduction to MRI for Medical Physicists and Engineers
Author: Anthony Wolbarst and Nathan YanasakISBN: 9781930524583
Published: June 2019 | 318 pp | eBook
Price: $ 120.00
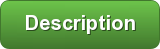
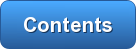
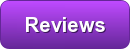
Table of Contents
Preface1. Introduction to MRI
1.1 A Stratospheric, Warp-Speed Sketch of NMR and MRI
1.2 Uniqueness of MRI
1.3 A Real MRI Case Study
1.4 A Brief History of MRI: Bloch, Purcell, Damadian, Lauterbur, et. al.
1.5 A Clinical Caveat
2. Quasi-Quantum, Two-State Picture of Proton NMR in a Single Voxel
2.1 A Brief Review of Electromagnetism
2.2 Magnetic Fields within Matter: Magnetic Susceptibility
2.3 Nuclear Magnetic Dipole Moment
2.4 Proton Spin-Up and Spin-Down States
2.5 NMR at the Larmor Resonance Frequency
2.6 Preliminary Demonstration of the NMR Phenomenon in Water
3. Proton Density MR Study of a 1D Multi-Voxel Patient and the Quality of the Image
3.1 The Three Distinct Applied Magnetic Fields in 1D MRI
3.2 Encoding of x-Position with an x-Gradient, and the Local NMR Resonance Frequency
3.3 Proton Density (PD) MRI of a Multi-Voxel, One-Dimensional Patient
3.4 Measures of Image Quality: Contrast, Resolution, and the MTF
3.5 Stochastic Noise, Signal-to-Noise Ratio, Contrast-Detail Curves, and the DQE
3.6 Judging Performance with ROC Curves
3.7 Some MR Artifacts
4. Magnetization of a Voxel
4.1 The Magnetization, m(x,t), of the Voxel at Location x
4.2 Thermal Equilibrium: The Battle between Energy and Entropy
4.3 The Magnitude of m(t) at Thermal Equilibrium, m0 ≡ m(∞), and Its Impact on Image Quality
4.4 Effect of Field Strength, |B0|, on Image Quality
4.5 Spin Population Dynamics
4.6 Stimulated Radiative Transitions and Spin System Saturation
4.7 Stimulated Non-Radiative Spin Transitions and T1 Relaxation
5. Mathematical Machinations
5.1 Two Simple but Essential Functions
5.2 Scalar (Dot) Product of Vectors and Functions
5.3 Fourier Series Expansion of a Function Periodic in Time or Space
5.4 Fourier Integrals and Transforms
5.5 Vectors in a 1D k-Space
5.6 Digital Representations of Symbols and Images
5.7 Noise, Statistics, and Probability Distribution Functions
5.8 Sampling an Analog Signal
5.9 Resolution vs. Detectability
6. Classical Approach to Proton MRI in a Voxel
6.1 Normal Modes of Oscillation
6.2 Resonance at the Normal Mode Frequency
6.3 In a Voxel, Classical Precession of m(t) about B0 at vLarmor; The Bloch Equations
6.4 Classical View of NMR
6.5 Grand Entrance of the 90° Pulse
6.6 Laboratory Frame of Reference vs. One Rotating at vLarmor
7. Free Induction Decay Imaging of a 1D Patient (without the Decay)
7.1 Saturation-Recovery in a Single Voxel, Assuming Unrealistically Slow Spin Relaxation
7.2 Improving the SNR with Quadrature Detection
7.3 Net Nuclear Magnetization from All the Voxels in the 1D Phantom Together
7.4 Selecting the z-Slice (or in 1D, the Row of Voxels) for Imaging
7.5 Signal from the 1D Phantom
7.6 FID Imaging of the 1D Phantom: Encoding Voxel x-Position Again with an x-Gradient
7.7 1D Image Reconstruction by Way of Frequency-Space
7.8 1D Image Reconstruction by Way of k-Space
7.9 Connections between Image Space and k-Space
7.10 Phase Waves
8. MRI Instrumentation
8.1 Main Magnet
8.2 Gradient Coils
8.3 The MRI RF System Is Like an AM Radio Transmitter/Receiver Pair
8.4 Antennae/RF Coils
8.5 Phased Array of Receiver Coils
9. T1 (Longitudinal, Spin-Lattice) Relaxation
9.1 The Longitudinal Proton Spin-Relaxation Time, T1, in a Single Voxel
9.2 Exponential Regrowth of mz(t) toward m0 at the Rate 1/T1: m(t) = m0 (1 – e(–t/T1))
9.3 The Bloch Equations, Including T1 Relaxation
9.4 Biophysical Mechanism of Proton T1 Relaxation: Spontaneous, Random, vLarmor Fluctuations
9.5 Tumbling Frequencies of Water Molecules in Three Aqueous Environments
9.6 T1 Increases with the Strength of the Main Field, B0
9.7 Determining T1 in a Voxel
9.8 T1 MRI in a 1D Patient
9.9 Speeding Things Up: Short TRs and Small Flip Angles
10. T2 (Transverse, Spin-Spin) Relaxation
10.1 Transverse (Spin-Spin) Proton Relaxation: Spin De-Phasing in the x-y Plane with Characteristic Time T2
10.2 Biophysics of Proton T2 Relaxation
10.3 Static Field de-Phasing, at the Rate 1/TSFd-P , and the Spin-Echo Sequence that Removes It
10.4 1/T2* is 1/T2 Speeded Up by 1/TSFd-P
10.5 Shape of an NM Resonance Line
10.6 Magnetization Transfer (MT) Imaging
10.7 Contrast Agents such as Gadolinium Chelates
11. The Spin-Echo Pulse Sequence
11.1 Spin-Echo (SE) RF Pulse Sequence in a Single Voxel: 90°–180°– read
11.2 Put Another Way…
11.3 And More Realistically…
11.4 The Spin-Echo RF Pulse Sequence Eliminates the TSFd-P Effect
11.5 Selecting TE and TR for T1-Weighted SE
11.6 Optimizing the T1 Contrast for a Pair of Nearby Voxels
11.7 T2-weighted and PD-w for SE
11.8 SE Reconstruction of the Image of a 1D Patient from a Single Line in k-Space
11.9 Dealing with Unwanted Phase Changes in the Echo Signal Caused by Gradients
11.10 Following a Simple Trajectory through 1D k-Space
11.11 Inversion Recovery (IR) in a Voxel: 180°–90°–180°– read
12. Image Reconstruction in Two Dimensions
12.1 Selection of a Two-Dimensional Slice
12.2 Waves in 2D Real Space and Vectors in 2D k-space
12.3 Phase Encoding of Voxel y-Position
12.4 A 2D, 4-Voxel Spin-Echo/Spin-Warp Example
12.5 The General Spin-Echo, Spin-Warp Echo Data Acquisition Process
13. Fast Imaging
13.1 Time to Acquire a Slice-Image
13.2 Multiple Slice Imaging
13.3 Stack of 2D Slices vs. 3D Block of Tissue
13.4 Fast Spin Echo vs. Conventional Spin Echo—More Readouts per Excitation
13.5 Gradient Echo (GE) / Gradient Recall Echo (GRE)—Reducing the Repetition Time (TR)
13.6 Echo Planar Imaging (EPI)—Many More Readouts per Excitation
13.7 k-Space Has (Approximate) Conjugate Symmetry, and its Data are Redundant
13.8 Under-sampling of k-space in Combination with Phased Array Coils
13.9 Frequency- and Phase-Encoding Similarities
13.10 Cartesian vs. Non-Cartesian Readout
14. Fluid Motion in One Dimension
14.1 Water and Blood Flow
14.2 Magnetic Resonance Angiography (MRA)
14.3 Perfusion Imaging
14.4 Diffusion Tensor Imaging (DTI)
14.5 Functional MRI (fMRI) with the Blood Oxygenation Level Dependent (BOLD) Effect
15. MRI Device Quality Assurance and Safety
15.1 Daily/Weekly/Monthly Quality Assurance Checks by Technologists, and the Semi-Annual QA by a Medical Physicist
15.2 Accreditation by the American College of Radiology Every Three Years
15.3 MR Safety Requires Eternal Vigilance (Regulations, Restricted Access, Records…)
15.4 Danger from the Main Magnet and Its Fringe Fields: Beware of Aneurysm Clips and Pacemakers,
15.5 Biological Hazards from Switching Gradients and RF Fields, etc.
15.6 Contrast Agents and Injectors
15.7 On Siting, Installing, and Accepting an MRI Device
16. On the Horizon: Imaging with a Crystal Ball
16.1 Evolution: Ongoing Changes
16.2 Revolution: Quantum Computers and Artificial Intelligence Quantum Artificial intelligence (AI)
16.3 Conclusion
Symbols and Abbreviations
Fundamental Equations
References and Sources
Index