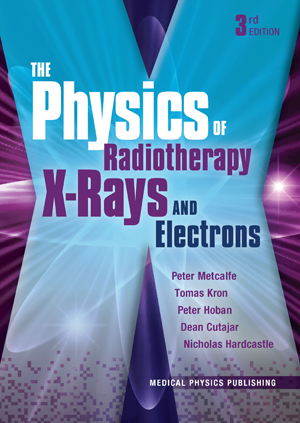
The Physics of Radiotherapy X-Rays and Electrons, 3rd Edition
Author: Peter Metcalfe, Tomas Kron, Peter Hoban, Dean Cutajar, Nicholas HardcastleISBN: 9781951134105
Published: March 2023 | 704 pp | Hardcover
Price: $ 185.00
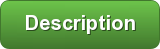
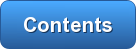
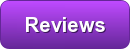
Table of Contents
Chapter 1: Medical Linear Accelerators
1.1 Introduction1.2 Principles of Linac Operation
1.2.1 External and Geometric Features
1.2.2 Operational Systems Overview
1.2.3 Auxiliary Systems Overview
1.2.4 The Modulator
1.3 Klystrons and Magnetrons
1.4 Electron Gun
1.5 Accelerator Waveguide
1.5.1 Traveling Waveguide Accelerator
1.5.2 Standing Waveguide Accelerator
1.5.3 Energy Selection
1.5.4 Electron Energy
1.6 Beam Delivery
1.6.1 Bending Magnet Assembly
1.6.2 Target and Flattening Filter
1.6.3 Monitor Unit Ionization Chambers
1.6.4 Electron Beam Delivery
1.7 Collimation
1.7.1 Primary Fixed Collimator
1.7.2 Secondary Collimators (Jaws)
1.7.3 Asymmetric Collimators
1.7.4 Blocks
1.7.5 Multileaf Collimators
1.8 Wedges
1.8.1 Physical Wedges
1.8.2 Flying Wedges
1.8.3 Dynamic Wedges
1.9 Image Guidance Devices
1.9.1 Electronic Portal Imaging Devices
1.9.2 On-Board kV Imaging and Cone-BeamCT
1.10 Innovative Linac Machines
1.10.1 Helical Tomotherapy-Radixact®
1.10.2 Halcyon-Ethos®
1.10.3 CyberKnife®
1.10.4 Hybrid MRI-Linac
Chapter 2: Interaction Properties of X-Rays and Electrons
2.1 Introduction2.2 Cross Sections and Attenuation Coefficients
2.2.1 Total and Differential Cross Section
2.2.2 Linear and Mass Attenuation Coefficient
2.2.3 Mass Energy Transfer and Absorption Coefficient
2.3 Photon Interaction Processes
2.3.1 Photoelectric Absorption
2.3.2 Compton (Incoherent) Scattering
2.3.3 Pair Production
2.3.4 Coherent Scattering
2.3.5 The Relative Importance of Each Process
2.4 Photon Beam Transport
2.4.1 Fluence and Attenuation of Polyenergetic Beams
2.4.2 Kerma, Collision Kerma, and Terma
2.4.3 Kerma and Dose for Different Energies
2.5 Electron Interactions
2.5.1 Collisional Energy Loss
2.5.2 Radiative Energy Loss (Bremsstrahlung Production)
2.5.3 Electron Stopping Powers
2.5.4 Electron Scattering Powers
2.6 Absorbed Dose
2.6.1 Dose, Kerma, and Charged Particle Equilibrium
2.6.2 Absorbed Dose from Exposure
2.7 Protons
2.7.1 Proton Interactions
2.7.2 Mass Stopping Power
2.7.3 Proton Range
Chapter 3: X-Ray Beam Properties
3.1 Introduction3.2 Dose Per Monitor Unit
3.3 Dose Build-up Region
3.3.1 Surface Dose and Skin Dose
3.3.2 Build-up Dose
3.4 Percentage Depth Dose
3.4.1 Definitions
3.4.2 Beam Energy
3.4.3 Field Size
3.4.4 Source-to-Surface Distance
3.5 Isocentric Dose Ratios
3.5.1 Tissue-Phantom Ratio
3.5.2 Tissue-Air Ratio and Scatter-Air Ratio
3.6 Beam Dose Profiles
3.6.1 Profile Shape
3.6.2 The Inter-Umbra Region
3.6.3 The Penumbra Region
3.7 Multileaf Collimators (MLCs)
3.8 Wedges and Dynamic Wedges
3.8.1 Physical Wedges
3.8.2 Dynamic Wedges
3.9 Monitor Unit Calculations
3.9.1 Calibration Position
3.9.2 SSD MU Calculations
3.9.3 SAD MU Calculations
3.10 Kilovoltage X-ray Therapy
Chapter 4: Linear Accelerator Electron Beam Properties
4.1 Introduction4.2 Characterization of a Clinical Electron Beam
4.3 Depth Doses and Profiles
4.4 Electron Applicators and Cut-Outs: the Practicalities of Getting Dose to the Patient
4.5 Physical Challenges in Electron Radiotherapy
4.5.1 Extended SSD
4.5.2 Small and Irregular Fields
4.5.3 Surface Dose and Bolus
4.5.4 Oblique Incidence
4.6 Inhomogeneities
4.7 Clinical Implications and Applications
4.7.1 Patient Contour Variations
4.7.2 Junctioning of Electron Beams
4.7.3 Electron Photon Combination
4.7.4 Electron Arcs
4.7.5 Total Body Electron Irradiation (TBEI)
4.7.6 Dealing with Shielding and Inhomogeneities
4.7.7 The Future of Electrons
4.8 A Few Comments on Protons
4.8.1 Proton Beam Production
4.8.2 Proton Beam Delivery to the Patient
4.8.3 Image Guidance and Range Uncertainty
4.8.4 A Note on Radiobiology
4.8.5 Clinical Experience
Chapter 5: Imaging for Radiotherapy Treatment Planning
5.1 Introduction5.2 Treatment Simulation Imaging
5.3 Computed Tomography Simulation
5.3.1 CT Scanners and Virtual Simulation
5.3.2 Obtaining CT Calibration Lines
5.3.3 Experimental Relation between CT Number and Electron Density
5.3.4 Theoretical Relation between CT Number and Electron Density
5.3.5 Dual-Energy and Spectral CT
5.3.6 Contrast CT
5.3.7 Four-Dimensional CT (4DCT)
5.4 Other Treatment Planning Aids
5.4.1 Magnetic Resonance Imaging
5.4.2 Nuclear Medicine Scans
5.5 Image Registration and Fusion
5.6 Conclusion
Chapter 6: Radiotherapy Treatment Planning: X-Rays
6.1 Introduction6.1.1 Definition
6.1.2 Steps in the Treatment Planning Process
6.1.3 Sections Included in This Chapter
6.2 Treatment Plan Prescribing and Reporting
6.3 Treatment Plan Geometry Definition
6.4 Creation of a Treatment Plan
6.5 Dose Display and Analysis Tools
6.5.1 Isodose Curves
6.5.2 Three-Dimensional (3D) Plan Displays
6.5.3 Dose Volume Histograms
6.6 Intensity Modulation
6.6.1 Forward and Inverse Planning
6.6.2 The Rationale for Intensity Modulation
6.6.3 Simple Optimization Mathematics
6.6.4 MLC Leaf Sequences for IMRT
6.6.5 Volumetric-Modulated Arc Therapy
6.6.6 Computational Approaches to Improve Inverse Planning
6.7 Dosimetric Properties of MLCs
6.7.1 Dosimetric Leaf Gap (DLG) and Radiation Field Offset (RFO)
6.7.2 Matchline Effects
6.7.3 Tongue and Groove Effect
6.7.4 Leaf Leakage
6.7.5 End Leaf Leakage
6.7.6 Complexity Metrics
6.8 Treatment Plan Quality Assurance
6.9 Summary
Chapter 7: Image-Guided Radiation Therapy and Motion Management
7.1 Introduction7.2 The ICRU Target Definitions
7.2.1 Irradiation Volume
7.2.2 Organs at Risk
7.2.3 Margins
7.2.4 Margin Recipes
7.3 Patient Setup and Immobilization
7.3.1 Patient Support Assembly: Couch
7.3.2 Immobilization Devices
7.3.3 External and Internal Markers
7.3.4 Developing Upright Setup Techniques
7.4 Inter-Fraction Motion Management: Image Guidance
7.4.1 MV Imaging
7.4.2 kV Imagers and Cone-Beam CT
7.4.3 Surface Guidance
7.5 Dose from Imaging in IGRT
7.6 Intra-Fraction Motion Management
7.6.1 4D Image Guidance
7.6.2 Physical Motion Reduction
7.6.3 Gated Treatment
7.6.4 Breath-Hold
7.6.5 Motion-Adaptive Therapy
7.7 Conclusion
Chapter 8: Adaptive Radiation Therapy (ART)
8.1 Introduction8.2 Principles (Why and What is Important)
8.2.1 Radiobiology
8.2.2 Time Scales
8.3 Technology for ART
8.3.1 ART on a Conventional Linear Accelerator (C-arm)
8.3.2 Dedicated ART Linear Accelerators with X-ray Guidance
8.3.3 Dedicated ART Machines MR-Linac
8.4 Anatomical ART
8.4.1 Concepts
8.4.2 Clinical Examples
8.5 Dose ART
8.6 Functional ART
8.6.1 Concepts
8.6.2 Clinical Examples
8.7 Artificial Intelligence for ART
8.8 Summary
Chapter 9: Stereotactic Radiosurgery
9.1 The Rationale for Stereotactic Radiosurgery9.2 Clinical Indications for SRS
9.3 Traditional Immobilization, Localization, and Targeting
9.3.1 The Head Ring
9.3.2 The Localizer
9.3.3 The Targeting Frame
9.3.4 Image Fusion
9.4 Frameless Techniques
9.4.1 Decoupling Immobilization and Localization
9.4.2 X-Ray and CT-Based Localization
9.4.3 Camera-Based Localization Using Infrared Markers
9.4.4 Camera-Based Localization Using Surface Imaging
9.5 Cone-Based Arc Beam Delivery
9.5.1 Plan Configuration
9.5.2 Dose Calculations
9.6 MLC-Based Beam Delivery
MLC-Based Arc Beam Delivery
9.7 Gamma Knife
9.7.1 The Perfexion
9.7.2 The Icon
9.8 CyberKnife
9.8.1 Beam Delivery
9.8.2 Image Guidance
9.8.3 Clinical Applications
9.9 Stereotactic Body Radiotherapy
9.10 ICRU Report 91
Chapter 10: Beam Models
10.1 Introduction10.2 Convolution/Superposition Methods
10.2.1 TERMA
10.2.2 Energy Deposition Kernels
10.2.3 The Convolution Process
10.2.4 Convolution in an Inhomogeneous Medium
10.2.5 Variation in Incident Beam Intensity
10.2.6 Variations of the Convolution Method
10.2.7 Differential Pencil-Beam Method
10.2.8 Collapsed Cone Convolution Method
10.2.9 Anisotropic Analytical Algorithm
10.2.10 Pencil-Beam Methods
10.3 Monte Carlo
10.3.1 Monte Carlo Principles
10.3.2 Random Number Generation
10.3.3 Statistical Uncertainties
10.3.4 Monte Carlo Particle Transport
10.3.5 Random Sampling from a Distribution
10.3.6 Variance Reduction
10.3.7 Monte Carlo Packages for Medical Radiation Physics
10.4 Monte Carlo Calculation Implementations for Treatment Planning
10.4.1 Electron Pencil Beams
10.4.2 Electron Monte Carlo
10.4.3 Photon Beam Monte Carlo
10.4.4 Photon Monte Carlo with GPUs (RayStation)
10.4.5 Monte Carlo Calculations for Protons
10.4.6 Monte Carlo Calculations for MRI-Linac
10.5 Linear Boltzmann Transport Equation
LBTE Numerical Solutions and Approximations
10.6 Dose to Medium
10.7 Clinical Impact of Dose Calculation Algorithm Variations
10.8 Brachytherapy Dose Calculations
10.8.1 Definitions
10.8.2 Types of Sources
10.8.3 The TG43 Formalism
10.8.4 Model-Based Calculations for Brachytherapy
10.9 Summary
Chapter 11: Dosimetry of Megavoltage X-Rays and Electrons
11.1 Why Measure Dose?11.2 The Ideal Dosimeter: Some Definitions
11.3 Radiotherapy Dosimetry Phantoms
11.3.1 General Considerations
11.3.2 Human Tissues and Phantom Materials
11.3.3 Water Phantoms
11.3.4 Solid Phantom Materials
11.3.5 Anthropomorphic Radiotherapy Phantoms
11.3.6 Phantoms and Motion
11.3.7 Customization and 3D Printing of Radiotherapy Phantoms
11.4 Ionization Chamber Dosimetry
11.4.1 Theoretical Background
11.4.2 Electron Equilibrium and the Free-Air Chamber
11.4.3 Bragg-Gray Cavity Theory and Chamber Correction Factors
11.4.4 Chamber Correction Factors
11.4.5 Practical Ionization Chambers for Radiotherapy
11.4.6 Electrometers
11.5 Silicon Semiconductors and Diamonds
11.5.1 Silicon Diodes
11.5.2 MOSFET Dosimeters
11.5.3 Diamonds
11.6 Luminescence Detectors and Scintillators
11.6.1 Thermoluminescence Dosimetry
11.6.2 Optically Stimulated Luminescence Dosimetry
11.6.3 Scintillators
11.7 Multi-Dimensional Detector Systems
11.7.1 Radiochromic Film
11.7.2 Detector Arrays
11.7.3 Electronic Portal Imaging Devices (EPIDs)
11.8 Other Dosimetry Systems of Interest
11.8.1 Calorimetry
11.8.2 Chemical Dosimetry
11.8.3 3D Gel Dosimeters
11.8.4 Biological Dosimetry
11.9 Reference Dosimetry
11.9.1 Choosing the Right “Kit” and Conditions
11.9.2 Characterization of Radiation Beams
11.9.3 Small Fields and Non-Reference Conditions
11.9.4 Uncertainty Analysis
11.9.5 Audits
11.10 Conclusion
Chapter 12: Quality Assurance in Treatment Delivery
12.1 Introduction12.2 Definitions and Dimensions of QA
12.2.1 Quality Management, Quality Assurance, and Quality Control
12.2.2 From Commissioning to QA
12.2.3 Action and Tolerance Levels
12.2.4 Accident Prevention and Quality Improvement
12.3 Patients and Machines, Technologies, and Techniques
12.3.1 Patient and Machine-Specific QA
12.3.2 Technologies and Techniques
12.4 Guidelines and Protocols
12.5 Risk Management
12.6 Practical Aspects of QA for Linear Accelerators
12.6.1 The Role of All Professions
12.6.2 Collaboration with Manufacturers
12.6.3 “Defense in Depth”
12.6.4 Expectations of QA, Costs, and Benefits
12.6.5 Research and Education
12.6.6 Documentation, Independent Review, and Audits
12.7 Conclusions
Chapter 13: Radiation Protection
13.1 Introduction13.2 Effective Dose (Sievert)
13.3 Background Radiation
13.4 Deterministic and Stochastic Radiation Effects
13.4.1 Deterministic Effects
13.4.2 Stochastic Effects
13.5 The Framework of Reports on Radiation Protection
13.6 Principles of Radiation Protection
13.6.1 Justification of Practice
13.6.2 The Optimization of Protection
13.6.3 Individual Effective Dose Limits
13.6.4 Types of Exposure
13.6.5 Effective Dose Limits
13.7 Practical Radiation Protection
13.8 Room Shielding Calculations
13.8.1 Primary, Secondary, and Leakage Radiation
13.8.2 Workload and Dose Limits
13.8.3 Shielding Calculations
13.4.4 Protection Against Neutrons
13.8.5 Radiation Surveys
Chapter 14: Tumor and Normal Tissue Response
14.1 Introduction14.2 Mechanism of Cell Killing
14.3 Introduction to Radiobiological Models
14.3.1 Nominal Standard Dose and Time Dose Fractionation
14.3.2 The Linear Quadratic Model
14.4 The Four Rs of Radiobiology
14.5 Linear Quadratic Model (Including Tumor Proliferation)
14.5.1 Biologically Effective Dose (Including Tumor Proliferation)
14.5.2 Accounting for Treatment Interruption
14.6 Models of Tumor and Normal Tissue Response
14.6.1 Functional Sub-Units (FSUs)
14.6.2 Inactivation of One FSU
14.6.3 Serial and Parallel Structures
14.6.4 NTCP for a Serial Organ
14.6.5 NTCP for a Parallel Organ
14.6.6 Tumor Control Probability
14.6.7 Use of TCP and NTCP in Treatment Planning
14.7 The LKB Model and Equivalent Uniform Dose
14.7.1 Generalized Equivalent Uniform Dose
14.7.2 The LKB Model
14.8 Multimetric Models
14.9 Clinical Trials Versus Model Prediction
14.9.1 Different Phase Trials
14.9.2 Different Levels of Evidence
14.10 Low Radiation Doses and Secondary Cancer Induction
14.11 Current Issues in Radiobiology
14.11.1 Low α/β Ratio and High Dose Per Fraction
14.11.2 Bystander Effect
14.11.3 Abscopal Effect
14.11.4 FLASH Radiotherapy
14.11.5 Metabolic Pathway Inhibitors